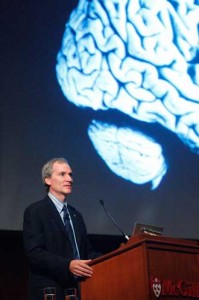
Beatty lecturer Dr. Marc Tessier-Lavigne explains neuroscience
By Neale McDevitt
Let’s get something straight: I am not a Science Guy, I am a Lit Guy. Rather than Gray’s Anatomy – the book – I cut my teeth on Walt Whitman’s Leaves of Grass, the poetry of the British Romantics and the barbed but beautiful prose of Charles Bukowski. In fact, ever since the miserable experience of Grade 10 biology, the closest thing I’ve come to any sort of meaningful scientific exploration is an abandoned Masters thesis on Mary Shelley’s Frankenstein.
Imagine my chagrin when I learned this year’s Beatty Memorial Lecture, which I cover every year, was going to be delivered by Dr. Marc Tessier-Lavigne, BSc’80, a world leader in the study of brain development and regeneration. The decidedly unpoetic title of Dr. Tessier-Lavigne’s lecture? Brain development and brain repair: The life and death of nerve cells.
So how is it that, 30 minutes into the Oct. 17 lecture, not only am I wide awake, I am also nodding my head vigorously and grinning like an I’ve-seen-the-light convert when Tessier-Lavigne says “we were able to pinpoint that the single protein called slit was responsible for the branching of axons”?
The answer is simple; a brilliant researcher who Medicine Nobel laureate Eric Kandel once called “the best that science can produce,” Tessier-Lavigne is as gifted in the classroom or at the podium as he is in the laboratory. No black arts were used to win me over, just good old-fashioned story-telling.
Tessier-Lavigne hooked me right away when he said that in its early stages, the embryonic brain has “a few hundred” or so nerve cells. But a mere nine months later, the newborn child has a trillion such cells, more intricately wired and interconnected than our most advanced computer. I can get that.
I then learn one of the building blocks of our central nervous system is the axon, a long, slender projection of a nerve cell, or neuron, that conducts electrical impulses away from the neuron’s cell body. Axons are in effect the primary transmission lines of the nervous system, and as bundles they help make up nerves. But how do these nascent cells, at their earliest stages of development, grow into such a complex system?
Tessier-Lavigne says that in a fetus’s early days, axons grow outward from the nerve cell like a dog on an ever-stretching leash, pulling its owner to a pre-determined destination.
But because some axons must make extremely long voyages to reach that final stop (the longest axons in the human body are those of the sciatic nerve, which run from the base of the spine to the big toe of each foot), the trip is broken up into smaller chunks – like pit stops on a cross-country car ride.
“Axons are guided by attractants and pushed away by repellents,” said Tessier-Lavigne, describing the specific molecules that keep the axon on its path. When it gets a whiff of something good, the dog pulls in the direction and, in contrast, will steer clear of bad smells – although, as owner of a couple of dogs himself, Tessier-Lavigne acknowledged the analogy’s inherent flaw because, “I’ve never known them to avoid any odour at all.”
But why don’t axons simply stop after they’ve reached one of their intermediate destinations? Because, we are told, axons are hardwired to be repelled almost immediately after hitting one of these mid-journey targets. “Reminiscent of some human relationships in which, sadly, initial attraction or infatuation can be destabilized by growing repulsion that that not only pushes the two people apart but can become intense enough that it actually erases all memory of the initial attraction,” joked Tessier-Lavigne. “Very much like serial monogamy.”
One of the most compelling possibilities for this type of research is its application in spinal-cord injuries. Tessier-Lavigne pointed out that, under the proper conditions, axons have an infinite capacity to regenerate. A damaged axon, severed from its final docking point, will almost immediately form a “growth cone” that starts pulling the leash in its effort to reconnect.
“Unfortunately [in the case of severe spinal-cord injuries], the environment is hostile to growth,” said Tessier-Lavigne, in part because the scar tissue associated with spine trauma contains Sema3F molecules – a known axon repellent. Looking to re-establish the broken circuit, the axon is driven back.
Tessier-Lavigne says the strategy for regeneration is to equip a damaged axon with “rose-coloured glasses” by switching off its Sema3F sensor. And while researchers are still a long way off in terms of human applications, experiments with lab mice have shown axons crossing over that great scar tissue divide.
“Hallelujah,” I think to myself, “and pass the Gray’s Anatomy.”