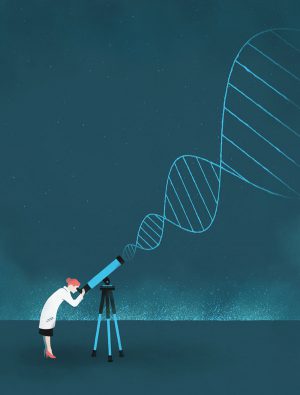
By James Martin
Many of the principal researchers in the McGill University and Génome Québec Innovation Centre came of academic age during the 13-year-long, multi-national Human Genome Project – but it’s the developments since the mapping of the human genome that fill them with wonder.
As a staff scientist at MIT in Boston, Ken Dewar worked in one of the many labs that collaborated on identifying the 3 billion molecules that, strung together in a double-helix, make up our DNA. Now an associate professor jointly appointed to McGill’s Department of Human Genetics and Division of Experimental Medicine, he still has a T-shirt from the big party in 1999 celebrating the sequencing of the genome’s first billion base pairs – less than a third of the way to the finish line. “It was a huge achievement,” he recalls. “But fast-forward to today, and it’s so completely trivial compared to what we can do now. It’s a totally different world.”

It took hundreds of scientists in dozens of labs more than a decade, and a billion dollars, to create that first “map” of all the cytosine, guanine, adenine and thymine nucleotide bases (abbreviated as simply, C, G, A or T) that chain together to create a single DNA molecule. Thanks to recent improvements in instrumentation speeds, that same result can now be achieved for a couple of thousand dollars in less than a week. In fact, today’s sequencing systems are so superior to that used in the Human Genome Project that it has become more efficient to sequence more than one genome at a time.
“In the last five years, the rate of production of genomic data has completely changed,” says Dr. Tomi Pastinen, who holds the Canada Research Chair in Human Genomics. Like Dewar, Pastinen works out of the McGill University and Génome Québec Innovation Centre. He remembers when a graduate student’s skill was measured in how deftly they handled a pipette; he estimates that 98 per cent of his research today takes place in front of a computer. In his own research, which focuses on diseases such as childhood leukemia, the recent technological advances have moved him away from hypothesis-driven experiments that focus on one or two genes at a time.
“It’s easier actually to study the whole genome at once, and then analyse data and extract what you need,” he says. “It’s more cost-effective to globally test everything, and it’s less biased toward what an individual investigator thinks might be important. In two weeks, you can generate enough data to keep you busy for two years.”
“But computer science has made amazing progress, too,” Dewar adds, “so we can do these analyses much faster than ever before.”
Delivering on the promise?
“Now the big question,” says his fellow centre researcher, Jacek Majewski, “is what do we do with all that data?” Majewski is an associate professor in the Department of Human Genetics and holds the Canada Research Chair in Statistical Genetics. He started his doctoral studies during the early days of the Human Genome Project, when DNA sequencing required toxic gels and jotting down long lists of nucleotides by hand. It was a labour-intensive process, but hopes were high for an eventual big payoff.
The hope was that those big diseases were each predominantly caused by a single, or at most a few defective genes. They’re not. Although there are rare forms of, say, diabetes that have been traced to a lone defect – and knowing the gene responsible can make it much easier to choose the appropriate therapy – the more common occurrences of these maladies are caused by the interactions of dozens of genes, not just a single misfire. “The person who is susceptible to diabetes probably has subtle variations in 10 to 20 genes, or even up to 200, that interact to cause the problem,” explains Majewski. “This makes it much harder to understand and target. The sheer complexity of the problem is not something that we foresaw when we started these projects.”

Dr. Rob Sladek, an endocrinologist and an associate professor of human genetics and medicine, echoes Majewski’s sentiments. “It’s true that complex and late onset diseases are far more complicated than we anticipated…but hope is far from lost. New methods and better-designed studies are making tremendous inroads.
“We’re moving into completely different realms of genomics, where our collaborations with groups in other fields, notably physics and computer science, are giving us the opportunity to monitor the dynamics of genome rearrangements and gene expression on single cells using nanotech devices.”
The legacy of the Human Genome Project is already paying dividends in basic research – and we’re already seeing that new knowledge is driving advances in clinical care. The effect, says Majewski, is most profound in two areas: testing for rare, recessive diseases and developing precision treatments for cancers.
As many as one in 20 people suffer from a rare disease that stems from congenital or metabolic defects. There are thousands such diseases, each having debilitating effects on the lives of a few hundred people. “These are things we can now prevent through genetic testing, and that’s thanks to the human genome. To me, that’s very exciting,” says Majewski. “For years, I was a basic researcher. I loved it, but it was very abstract. Now having something that’s able to help somebody is a gratifying part of the research.”
Genomics is also proving the key to getting cancer patients started on the correct drugs much earlier in their treatments than ever before. By sequencing a biopsy, and comparing a cancerous tumour’s molecular profile against a database showing how certain mutations have responded to particular drug therapies, a physician can now more quickly prescribe the best treatment. One of the cancers that Majewski studies is glioblastoma, an aggressive brain tumour that might be caused by a chromosomal rearrangement. Basic genomic research has revealed that this rearrangement fuses two gene products (FGFR and TACC); a new drug treatment that inhibits FGFR function is now showing promise in treating glioblastoma patients.
What’s next?
Although precision therapy already has profound implications for improving patient outcomes after disease has struck, Dewar isn’t sure genomics will ever yield the Holy Grail: tweaking our genes to prevent disease from ever starting. “There are tremendous hurdles,” says Dewar, whose two main research streams focus on monkeys and microbes. “Count the number of cells in your body that have a nucleus. Every nucleus has a copy of your genome – and you have to find a way to correct them all? It’s unclear how realistic that will ever be. Instead, we need to find out how to counteract the defect by other means.”
But if modifying every copy of a person’s genome isn’t feasible, modifying the microbiome, the collective genome of all the microorganisms living inside that individual, is one of the possible avenues. Dewar led the team that sequenced the strain of C. difficile that killed hundreds of people in Quebec during a 2003 outbreak; sequencing those bacteria has allowed better testing to identify patients infected with the most virulent strains. He points to the 95-per-cent success rate of fecal-transplant therapy in curing C. difficile, where traditional antibiotics have failed. (Fecal-transplant therapy is exactly what it sounds like: curing a sick person by recalibrating their micro-ecology through controlled exposure to the feces of a healthy, non-infected donor.) Dewar suspects that this kind of literal micromanaging of the genetics of the microbiome might prove the path to eliminating, or controlling, many illnesses in the near future. Possible candidates for this treatment include disorders related to nutrient uptake and metabolism, as well as bowel inflammation syndromes.
“Those studies of microbial ecologies and populations could not have been considered 10 years ago because of the complexity of the number of species and the cumulative size of the genome of all the things in there,” he says. “But now, we can sequence them – the problem is figuring out what exactly we’ve sequenced when 90 per cent of them are completely new to science.”

“Things have moved so fast,” says Pastinen. “The more we do genome science, the more granularity we find in the way human genomes and human cells work. Even though I worked on genomic techniques during the later stages of my MD/PhD, and knew what was coming, I couldn’t have envisioned what happened in the past 10 years.”
Confident that cancer genomics has revealed “almost all the genetic culprits” that disrupt the epigenome (the genome’s chemical functions), Majewski’s and Pastinen’s teams are now collaborating with basic researchers at Rockefeller University to design new molecules to repair the problem.
“I love the fact that the two research approaches are coming together,” Pastinen says. “The basic researchers have been studying these epigenetic processes for many years. And human mutations link them to direct phenotypic consequence: They cause cancer. Now, on the applied side, we can study it backwards to understand how these mutations disrupt the basic process, then design new drugs to counter those defects.
“My basic training is in medicine,” he adds. “My interest is still in improving the human condition, not just in finding out how the genes turn on and off. If you just want to understand biology, you can do it in yeast.”
The research described in this article is funded by the Bachynski Family Foundation, the Canada Foundation for Innovation, the Canadian Cancer Society Research Institute, the Canadian Institutes of Health Research, the Cancer Research Society, the Crohn’s and Colitis Foundation Canada, the Fonds de recherche du Quebec – Santé, Genome Canada, Genome Quebec, the National Institute of Diabetes and Digestive and Kidney Diseases, the National Institutes of Health and Tekes (Finland).