by Mark Reynolds
Tiny technology promises a fix for broken nerves
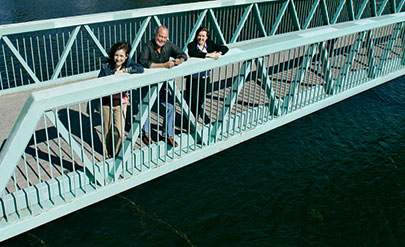
Anna Lisa Lucido, a PhD student at McGill’s Montreal Neurological Institute (MNI), is visibly excited. It’s late afternoon in the office of her supervisor, MNI director David Colman, and she is presenting experimental results that are, in her words, “hot off the microscope” from that morning.
On her laptop screen are digital images that look like stills from The Blob. An organic mass, splotchy and irregular in shape, extends tendrils and bulging protuberances as if to absorb the smooth and evidently man-made orb that dominates the right side of the image.
“Pretty neat, eh?” she says with a grin.
Neat indeed – it could represent the birth of a whole new way of doing medicine. The orb is a latex bead, micrometres in diameter, coated with the organic compound polylysine; the organic blob is a neuron. Lucido and Colman, a Canada Research Chair, believe the image captured on that slide was the cell attempting to form a synapse—an active communication—with the bead.
Get that cell communicating with some man-made electronics, and it could be possible to create a bridge between severed nerves and muscles up to a metre away—light years in neuronal terms. The possibilities are breathtaking—malfunctioning glands linked to artificial regulators, severed spinal cords repaired, stroke victims rehabilitated.
Not Science Fiction
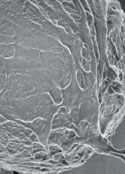
Scanning electron micrograph of a single latex bead (above), which has attracted neurons to extend processes (or neurites) over its surface. Some of these processes will later form presynaptic contacts with the beads.
Lucido’s slides stem from a collaboration between scientists and researchers at the MNI and the McGill Institute for Advanced Materials (MIAM) that brings the science of the very small to bear on age-old medical problems.
Nanoscience, which operates at a scale of billionths of a metre, still has a whiff of science fiction about it. But Colman, who originated the MNI’s Program in NeuroEngineering, which recently received a Canadian Institutes of Health Research (CIHR) grant, has faith it will soon be reality.
“I’m sure it’s the field of the future,” he says, while acknowl- edging many high-profile developments in nanoscience are novelties.
“Most of the nanotechnology that the public sees is, unfortunately, the gimmicky things like tiny nano-guitars or robots that will get the parasites off of your lawn—this stuff is just silly.”
According to Colman, the real benefits of nanotechnology will come at the molecular level. At that scale, the difference between living and non-living becomes less a clear division than a spectrum: a hydrocarbon molecule is a hydrocarbon molecule whether part of a living cell or a plastic nano-widget. The challenge of the collaboration Colman has set in motion is to get the hydrocarbons from the living system to interact with the hydrocarbons from the man-made device.
Bridging the Gap
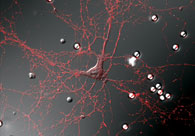
First, though, they have to get the two systems together. Lucido’s blob in a petri dish would not have grown toward the bead in a live body—as Dr. Alyson Fournier explains, the central nervous system releases chemicals that stop neurons from growing. In a living system, this makes sense; nerves can’t be left to grow willy-nilly. But it means when they’re injured, there is no recovery—yet.
According to Fournier, a Canada Research Chair and professor of neurology and neurosurgery at the MNI, overcoming the body’s inhibitory system will be key to regrowing nerves, either to reconnect them over short distances or to attach them to an artificial matrix as Colman envisions. Working with chemistry professor Christopher Barrett, Fournier is looking to create a bridge—a substrate—that will encourage a nerve axon to grow, and determine in which direction.
“This way, you could imagine guiding an injured nerve fibre a short distance to an artificial device and then linking it to the original connection with artificial fibres. But to do that, we need to be able to artificially promote nerve fibre growth in a targeted and dynamic way following an injury,” she says.
The “targeting” part of that is key. To be useful, the nerve has to grow to an exact location—within a few microns—to form a synapse. The substrate Fournier is developing with Barrett will be photosensitive—shine a light on it, and you change its properties. This means that a cell’s growth could be precisely directed.
Collaboration, not Coffee
To set the stage for targeted nerve regrowth, Colman had to first integrate the disciplines of medicine, engineering, chemistry, physics and computer science. Early attempts were plagued by miscommunications.
“The biologists would get up and say, ‘Here’s what we do,’ and the physical science people would say, ‘Here are the tools we have,'” recalls Colman, adding wryly, “Then there would be coffee hour.”
Coffee was fine, but Colman wanted collaboration. The joint project finally got off the ground when Colman, who describes himself as a biologist who happens to work on neurological problems, encouraged people not to present their research, but their goals, about which other scientists might be able to feel excited.
Enter MIAM member and Chair of the Chemistry Department Bruce Lennox, whose lab is examining the chemistry of the polylysine-bead matrix.
“Chemistry interfaces very well with biological sciences,” he says. “We often have molecules that we want to apply in other fields—in other words, we have solutions looking for problems.”
Lennox has been working with biological scientists at various levels of collaboration for much of his 15 years at McGill. He is particularly enthused about the project between the two institutes, largely because of Colman’s approach of ensuring that all participants are getting something out of the exchange.
“I think interdisciplinary research is very powerful when someone like myself, a chemist, can find high-end chemical problems in someone else’s research. I’m not just repeating what we’ve done before—and that’s when everybody wins.”
Beyond our Imagination
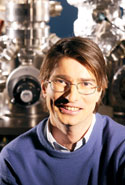
“Everybody” includes Peter Grütter, a MIAM member, physicist and Scientific Director of the Natural Sciences and Engineering Research Council of Canada’s (NSERC) Nano Innovation Platform, which coordinates nanotechnology across the country. Grütter provides expertise in atomic force microscopy (AFM), an imaging, measurement and manipulation technology that exploits the forces inherent in all materials. AFM can “see” and move objects at the molecular level, even detecting changes in neurons as they process signals.
Though Grütter is delighted to play a part in solving the medical riddles faced by MNI doctors, he sees in the unique collaboration a chance to investigate the possibilities of new computing technologies developed on the model of the human nervous system—not to mention access to CIHR funding to complement his existing NSERC grants. Perhaps reflecting his years at IBM prior to coming to McGill, Grütter speaks of Mother Nature as a rival engineer with a “killer application” that he’d like to further develop.
Grütter is particularly intrigued by the nervous system’s ability to stop signalling information when it isn’t important, a capability that exists at the “low” level of individual synapses, not the brain. In Grütter’s words, this allows the “central processor” (the brain) to not be so overwhelmed by, for example, a swarm of mosquitoes that it misses an attacking lion.
“If you can understand how this works, you might be able to reverse engineer a similar architecture with different materials,” he says. “Neurons are a proof of principle that you can build extremely powerful processors—like our brain—on a totally different architecture than a computer.”
New computers were not what Colman envisioned from the collaboration between the two McGill institutes, but he’s fine with that. “A lot of very imaginative people are going to come up with things that I can’t think of now. The applications of this can far exceed what we can imagine.”
The Program in NeuroEngineering is funded by the Regenerative Medicine and Nanomedicine initiative of the Canadian Institutes of Health Research.