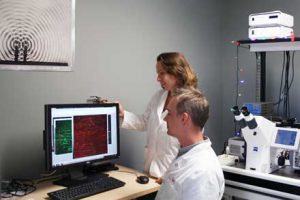
Research into protein molecule sheds new light on how brain cells construct their internal ‘skeletons’
By Chris Chipello
In a corner of a second floor laboratory in the Bellini Life Sciences Complex, a research team led by Department of Biology Prof. Gary Brouhard and postdoctoral fellow Susanne Bechstedt has spent much of the past two and a half years scrutinizing the behaviour of a protein known as doublecortin.
The result of their painstaking observations, published recently in the journal Developmental Cell, is the discovery of the cause of an inherited form of epilepsy called double-cortex syndrome.
Scientists have understood since the 1990s that double-cortex syndrome, which primarily affects females, stems from inherited mutations on a gene located on the X chromosome. Production of doublecortin is regulated by that gene, known as the DCX gene.
As the brain develops in the uterus, new brain cells are born deep within the brain. These newborn cells then crawl out of the “niche” where they were born and migrate outward to the edges of the brain. This outermost layer of the brain, known as the cerebral cortex, is the seat of all higher-level thinking and cognition.
In girls with a mutation in their DCX gene, the outward migration of brain cells unfortunately fails. Instead of making it all the way to the edges of the brain, some of the cells pile up and form a secondary or “double-cortex.” The activity of these abnormally placed cells gives rise to seizures and also, in some cases, mental retardation.
But exactly how the gene mutation caused this anomaly wasn’t clear – until now.
To find the answer, Bechstedt and Brouhard began by purifying the doublecortin protein and watching it under a microscope equipped with a highly sensitive digital camera. The powerful instrument enabled them to observe individual protein molecules – each less than 10 nanometers in size – in action.
The doublecortin protein helps brain cells to build inside themselves a scaffold, much like those at construction sites, made of “poles” called microtubules. These form a structural framework for brain cells, known as the cytoskeleton. Brain cells require this internal skeleton to crawl and to migrate, much as humans need their skeletons to walk and run.
As they monitored the protein, Brouhard and Bechstedt discovered that, in order for doublecortin to help build this scaffold, many doublecortin proteins must work together as a team. They also found that disease-causing mutations caused a breakdown in teamwork, preventing the brain cells from constructing a proper “skeleton.”
“Once we had purified the protein, the rest went relatively quickly,” Brouhard says. Because patients with double-cortex syndrome have a variety of different inherited mutations, “it’s as if nature has run a genetics experiment” that scientists can draw on. “If you’re working on a protein that has no disease link, you have to introduce mutations at random and hope that they tell you something interesting,” he explains. “But with these patients we had a library of mutations that we knew had an effect in human-brain development. That made it simple for us to introduce those mutations into our protein and to then test the effect of those mutations in our experiments.”
Brouhard and Bechstedt met at the Max Planck Institute of Molecular Cell Biology and Genetics in Dresden, Germany, where Brouhard did his postdoctoral work after completing his Ph.D. at the University of Michigan in 2004. After Brouhard joined McGill as an assistant professor in 2008, Bechstedt – who was a grad student at the Planck Institute – applied to join his lab as a postdoc. It was Bechstedt’s idea for the lab to look specifically at the doublecortin protein, Brouhard says.
The resulting findings have potential implications for treatments for a range of conditions, from other forms of epilepsy to spinal cord injuries. Improved understanding of how brain cells construct their skeletons could open avenues for doctors to target the cytoskeleton to extend and re-grow when needed. Scientists already are exploring ways to use gene therapy for that purpose – though in most cases such techniques remain a distant vision, Brouhard cautions. A more likely approach in the shorter term, he says, is for drugs to be applied to specific injury conditions, such as spinal cord wounds, to stimulate microtubule activity and prompt regrowth of nerve cells.
For their part, Brouhard and Bechstedt plan to turn their attention to a couple of important questions that have emerged from their basic research into doublecortin.
“We have a few patient mutations that – unlike the ones we published – had almost no negative consequences in our experiments,” Brouhard says. “They bound to microtubules really well.” In these patients, the “broken” element of the molecule was a tail-like appendage that the cell uses to turn on or off doublecortin – leaving it essentially “on” at all times. “What we don’t understand is when and how cells need to turn doublecortin on and off. We’re very interested in how doublecortin is controlled – how cells turn it on and off.”
In addition, “there are 11 genes similar to doublecortin in humans – several of which have been implicated in other types of disease,” including dyslexia and an eye condition called retinitis pigmentosa. “We plan to look at whether or not some of the things that we’ve learned about doublecortin are conserved” throughout the entire family of related genes. “Do they all bind to microtubules in similar ways? And in the diseases like dyslexia and retinitis pigmentosa, what are the defects that are causing those conditions?”
The research reported by Brouhard and Bechstedt in Developmental Cell was funded by the Canadian Institutes of Health Research. The article is available here.